When you are to invest in a new QCM system, it is relevant to compare similar technologies as well as to evaluate QCM instruments from different suppliers to make sure you find a good fit for your lab.
Comparing QCM-D to other surface sensitive technologies
QCM-D is often compared to other surface sensitive real-time technologies, such as SPR and ellipsometry, which also provide information on molecule-surface interactions. So how does QCM-D relate to these two methods?
QCM-D vs SPR
QCM-D and Surface plasmon resonance (SPR) are similar in many ways. For example, both methods are used in surface interaction analysis to monitor specific interactions, molecular binding, and adsorption events. As the two methods are based on different technologies and measurement principles, however, QCM being an acoustic technology and SPR being an optical one, there are differences in experimental capabilities and information extraction.
As already discussed, QCM measures the resonance frequency changes, f, of an oscillating quartz crystal which is sensitive to mass changes at the surface. SPR, on the other hand, measures change of the surface plasmon resonance angle, ϴ, which is sensitive to refractive index changes. In addition to f and ϴ, some QCM and SPR instruments are designed to capture additional parameters, which then expands the amount of information that the respective technology can extract. The information output offered by a QCM instrument ranges from qualitative information on time-resolved mass changes at the surface, to quantitative information about mass, thickness and viscoelastic properties at high time resolution. The information output offered by a standard SPR instrument ranges from relative information about refractive index changes at/ near the surface, to quantitative information about the refractive index, thickness, density, and surface coverage.
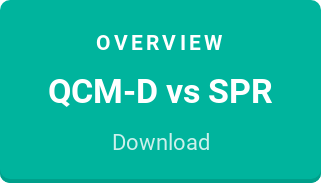
QCM-D vs Ellipsometry
QCM-D and ellipsometry also show many similarities. Both technologies provide real-time results on surface adsorption and desorption and allow for quantification of the surface mass density of layers at the solid-liquid or solid-gas interface. The operating principles of the two methods are however different; QCM measures acoustic changes, i.e., changes of a sound signal, whereas ellipsometry measures optical changes, i.e., changes of a light signal.
As already mentioned, both technologies are able to sense mass changes at the surface. Due to the differences in the working principles, however, the mass that is sensed differs between the two methods. The mass sensed by QCM technology includes not only the molecules adsorbed/bound to the sensor surface, but also the mass of the solvent associated with the adsorbed layer. Ellipsometry, on the other hand, measures mass excluding the associated solvent. This is the reason why optical mass is often referred to as “dry mass” and acoustic mass often referred to as “wet mass”. Since the two methods offer complementary information, using a combined setup, where QCM-D and ellipsometry can be run simultaneously on the same surface, makes it possible to extract information on variations in the amount of coupled solvent in the system under study, which is information that neither of the two techniques is able to extract when used on its own.

QCM-D vs other QCM:s
There are a number of different QCM:s available in the market - standard QCM:s, which only measure the resonance frequency, extended QCM:s – which in addition to the frequency also measure the energy loss, single-harmonic QCM:s which typically only measure at the fundamental resonance frequency, and multi-harmonic QCM:s – QCM:s which measure at multiple harmonics. In addition to this, several aspects of the instrument specifications, for example the fundamental resonance frequency, can vary between the instruments.
Standard QCM
In brief, standard QCM measures changes in resonance frequency of the quartz crystal upon excitation by a driving voltage. This measurement allows for monitoring of changes in mass or thickness of thin and rigid layers adhering to the surface of the quartz crystal sensor. If the adsorbed (or bound) layer obeys the requirements of the Sauerbrey relation, information about mass and thickness of the layer can be extracted.
Extended QCM - QCM that monitors the energy loss
Instruments such as QCM-D, QCM-I, QCM-R, etc., are extended versions of the standard QCM. In addition to measuring changes of the resonance frequency, f, these QCM:s also measure changes of the energy loss, the dissipation.
In brief, there are three different ways to measure the energy loss - via 1) impedance spectroscopy, via 2) the decay time of the oscillation, or 3) by measuring the resistance. The various approaches provide different amounts of information about the system under study. Thanks to the additional inormation provided by the extended QCMs, it is for example possible to reveal whether the measured layer is rigid or not, and if the Sauerbrey equation can be used for the quantification of mass.
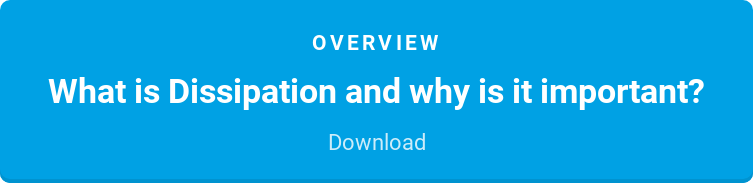
Single-harmonic vs multi-harmonic QCM
Like a guitar string, a QCM crystal can be excited to resonate at several different harmonics. A single harmonic QCM excites the crystal at one frequency whereas a multi-harmonic QCM excites the crystal at multiple frequencies. The number of frequencies measured by a multi-harmonic QCM can vary from two and up. Single harmonic QCMs typically use the fundamental while multi-harmonic QCMs use the fundamental frequency and one or several overtones.
Each harmonic measured provides information about the system under study. If f and D are collected at multiple harmonics, it is possible to quantify mass, thickness and viscoelastic properties of soft layers, i.e. multi-harmonic QCM-D technology enables quantification of mass and thickness of rigid as well as of viscoelastic layers.
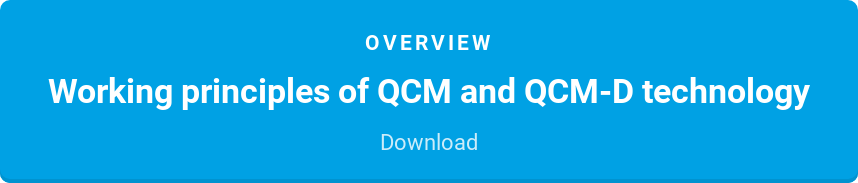
Which QCM will suit your needs?
Each of the QCM-versions here discussed are suitable for different types of measurements and experimental contexts. Which QCM you should go for therefore depends on your specific application and how carefully you need to characterize the system that you plan to work with. Key questions to ask are:
- Will measurements be taken in the gas phase or in the liquid phase?
- Will viscoelastic layers be studied?
- Will the processes studied be fast or slow?
- Is quantitative information needed, or will qualitative information be sufficient?
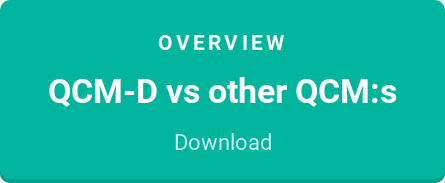
How to assess and compare QCM-specifications
Once you have decided which type of QCM instrument you need, and have identified possible suppliers, it is time to compare the specifications for the different instruments that you are choosing between. Assessing and comparing QCM specifications can, however, be tricky, as they do not always include the same information, and the terminology used to describe a certain property can differ. One approach to facilitate the comparison of the information provided in the specificatrions is to identify the parameters that are relevant to the actual measurement situation, and to look at the unit of the specified numbers to compare the information between different suppliers.
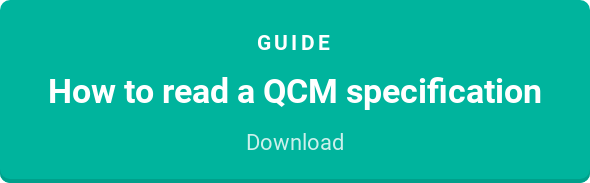
If you are interested in a QSense instrument, have a look at our full range of products, try our instrument selector, or reach out to us to start a conversation about your research needs.